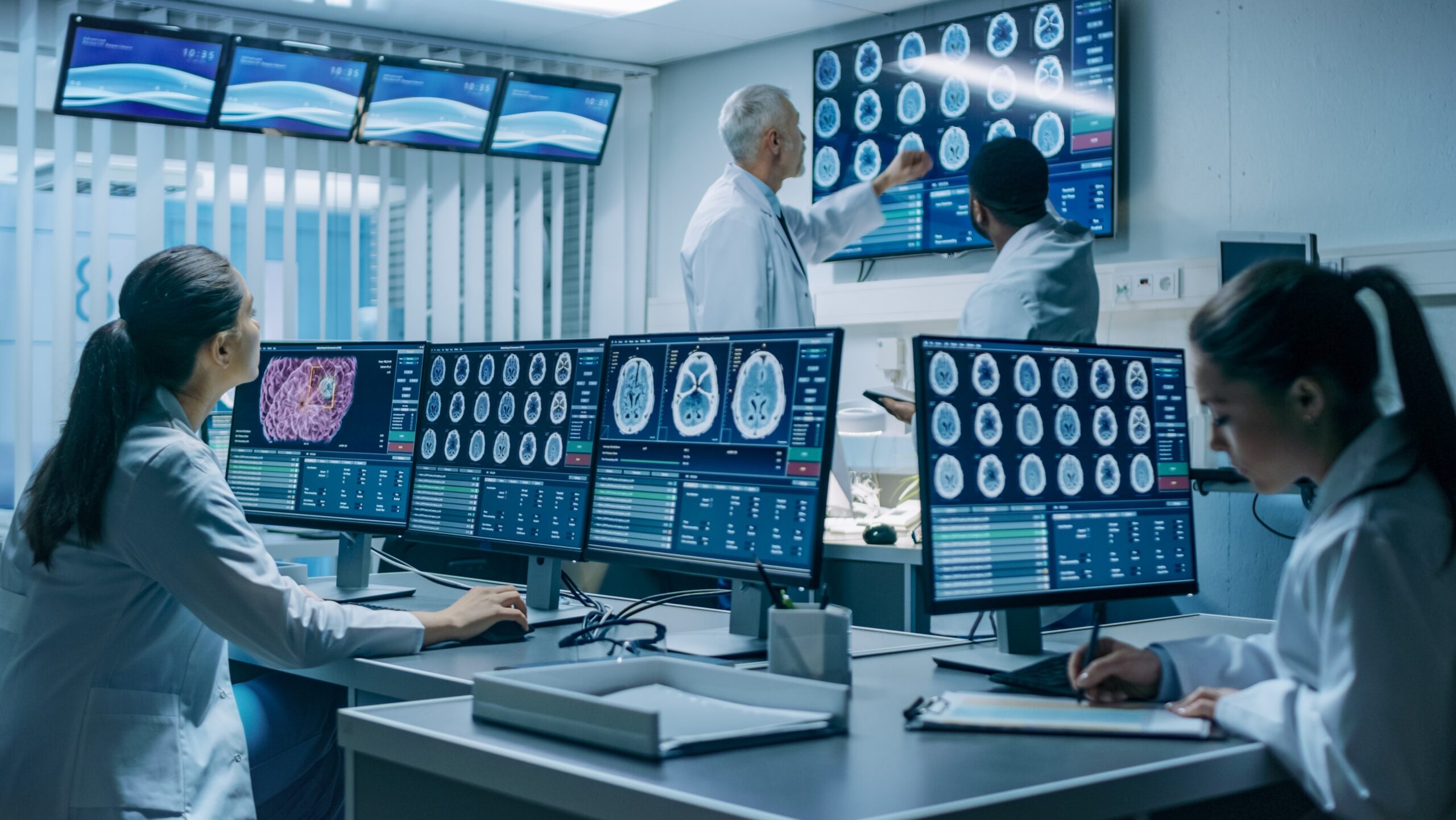
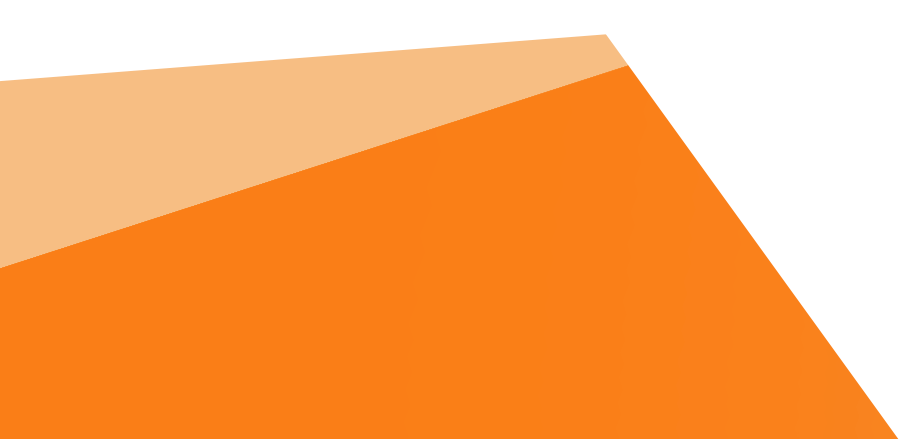
Precision I/O Clinical Trial: Leveraging Technology to Gain Insight into Treatment Efficacy
Cancer treatment is reaching a breakthrough due to the advances in Immunotherapy. The fundamental technology behind immunotherapy is to harness the body’s own immune system to attack cancer cells. The original purpose of immune cells is to identify and destroy dangerous cells. However, cancer cells have developed the ability to hide from the immune system and therefore uncontrolled cell proliferation occurs which leads to tumour growth. In principle, immunotherapy makes cancer cells visible to the immune system. Therapies address both: They target the body’s immune cells to identify cancer cells and enable them to destroy the dangerous cells. Therefore, immunotherapies are also called targeted therapies.
For the first time in cancer treatment it is possible to address tumour heterogeneity in a more specific way. Immunotherapy enables us to study and manipulate the tumour microenvironment. However, to evaluate, proof and leverage the potential of immunotherapies, it is essential to find methods which can show the changes in the tumour microenvironment non-invasively.
This is where advanced medical imaging followed by computer-based analysis becomes paramount. Advanced magnetic resonance imaging (MRI) in combination with algorithms to calculate quantitative imaging biomarkers offer the unique opportunity to provide sensitive and specific tumour measurements. Especially in clinical research studies which aim not only to test the safety of a drug but also the efficacy in a certain patient population, the sensitive assessment of the therapy induced tumour microenvironmental changes are crucial for bringing the drug to the market.
Immunotherapy: Harnessing the Immune System
The immune system is the body’s natural defence system, attacking dangerous cells and destroying them. Cancer cells have developed the ability to hide from the immune system and thereby prevent an immune response. Due to their inherent mechanism of action, immunotherapies are also called targeted therapies or combination therapies. The first part of the therapy is to target the immune system to attack the cancer cells systematically: The immune cells (T-cells) are directed in the body towards the tumour; T-cells need to be close to a cell to test if it is dangerous. Second, immune cells are empowered to see and destroy the cancer cells. Making dangerous cancer cells visible to T-cells is the task of drugs known as immune checkpoint inhibitors.
T-cells must be close to cancer cells to enable the checkpoint antibodies to reveal the cancer cells and fight them.
Cancer in Detail: The Tumour Microenvironment
The tumour microenvironment of many tumour types, such as glioblastoma (GBM), the aggressive and most common form of brain cancer, is heterogeneous. This means the tumour is composed out of different cells types. The efficient treatment of GBM is still an unmet clinical need. Much research is focused on GBM treatment development: Multimodal therapies (including radiation, chemo and surgery) as well as exciting novel approaches such as Immuno-Oncology therapies, are constantly being developed and tested.
The complex nature of the tumour itself requires not only more advanced therapies but also better insights and more advanced methods to proof therapy efficacy.
To understand a therapy’s mode of action and evaluate treatment efficacy, morphological as well as functional details of the tumour changes need to be not only visualised but also measured.
Immunotherapies cause different morphological and functional tumour changes than conventional therapies. The tumour might not shrink but just stop growing. Complex, non-uniform tumour shrinkage has been observed. Metabolic activity can even lead to an initial increase in the size of the tumour. This effect is called pseudo-progression. The key here is to be able to differentiate pseudo-progression from true progression.
Visualising and Measuring: the Contribution of Advanced Imaging
Magnetic Resonance Imaging (MRI) is a non-invasive imaging technique based on a signal that is send out from water molecules in a strong magnetic field. Over the years many different MRI sequences have been and still are developed. Different MRI scanner sequences focus on different tissue characteristics. Conventional and well known sequences (T1-weighted or T2-weighted MRI) focus on anatomies such as bone, muscle, fat or cerebrospinal fluid (CSF). Conventional radiographs or computed tomography (CT) are anatomical sequences too however, MRI provides more detail for soft tissue.
MRI physicists have further exploited the potential that lies within MRI scanners. Based on the scanner’s sensitivity to depict water-based structures, they introduced functional MRI sequences. Popular examples of functional sequences are Diffusion Weighted Imaging (DWI) and Dynamic Contrast Enhanced (DCE) MRI. DWI measures the movement of water molecules in tissue. This sequence is used to reconstruct neuronal pathways in the brain but also to measure the cellular density. DCE-MRI uses a gadolinium enhanced contrast agent that is injected in the blood stream during image acquisition. The contrast agent changes the signal intensity and therefore makes blood visible in the image. This is particularly beneficial in inflammatory or necrotic tissue.
Since MRI settings are non-invasive and novel scanners allow fast imaging, multi parametric MRI (mpMRI) became feasible. mpMRI is a combination of anatomical and functional sequences within one MRI setting.
This allows to assess functional characteristics such as tumour perfusion and cellular density alongside anatomical high resolution lesion location.
To ensure highest sensitivity and specify, the MRI protocol must be carefully designed for each therapeutic area, clinical question and applicable in a multi-center clinical trial environment. Various pitfalls such as spatial and temporal resolution, multi-vendor scanner protocols and scanner parameter exist.
Enhanced Sensitivity: the Impact for Drug Development
Clinical trials in cancer drug development must proof the safety and efficacy of immunotherapies in certain cancer types. This is challenging in multiple ways: finding the right patients, designing a study with adequate methods to show efficacy, aligning with regulatory requirements as well as finding investors for the study.
Conventional approaches in oncology clinical trials aim to show drug efficacy based on the assumption that a tumour shrinkage is significant for the assessment of the change in tumour burden. Tumour shrinkage is conventionally measured using anatomical imaging modalities such as CT or MRI in combination with the Response Evaluation Criteria In Solid Tumors (RECIST)1,2-based scoring methods. RECIST is a diameter based assessment in which a reader (board certified radiologist) defines the disease burden by measuring the size of a lesion in an image.
Even though diameter-based methods such as RECIST, have their place, for example in late stage clinical trials where the mode of action and drug efficacy has been understood and shown, they are not adequate to capture complex tumour microenvironmental changes. Particularly studies in advanced therapies, such as immunotherapy, or in heterogeneous tumours, such as glioblastoma, are prone to error when endpoints are used that assume tumour shrinkage.
Immuno-therapy for example has shown to lead to the so called pseudo-progression; the initial increase of tumour burden followed by a later treatment response. The phenomena are called pseudo-progression, because per RECIST they would be classified as progressive disease. Recently, RECIST has been updated to RECIST1.14. The major changes are the number of lesions to be assessed and the assessment of pathological lymph nodes.
However, RECIST1.1 still does not account for immuno-therapy related changes. Several updates considering the potential false evaluation of pseudo-progression emerged: immune-related response criteria (irRC)5, which is based on the WHO criteria, Immune-related RECIST (irRECIST)6, a combination of irRC and RECIST and Immune RECIST (iRECIST)7, a standardised, evaluated version for use in clinical research.
All RECIST amendments are valuable and useful for drug efficacy evaluation. However, they are not sensitive enough to provide more detail into therapy induced tumour microenvironmental changes early and with scientific accuracy.
Applying less than adequate image analysis methods in clinical trials has not only the danger of keeping cancer patients on ineffective treatments for too long, waiting for a potential later response after pseudo-progression, but wasting precious time, investment and R&D resources without getting the right answers. Besides extending the trial unnecessarily, the question is if the applied methods are at all able to show the drug induced changes in the tumour.
Adequate scientific methods must be applied to enable the clinical trial to reveal the drug efficacy.
Leveraging Technology: the Insight that Advanced MRI Offers
CT or MRI are routinely used in the initial evaluation of tumour size, shape, and anatomic appearance. We believe that in early efficacy trials, it is paramount to extend the imaging protocol with the sequences that allow to incorporate the measurements (biomarkers), which adequately reflect therapy relevant changes. Such biomarkers should guide our quantitative and objective assessment of the drug effect and overall decision about the early efficacy of novel treatments.
The most relevant sequences for immuno-oncology related drug development are: functional MRI sequences, including Dynamic Contrast Enhanced (DCE)-MRI and Diffusion Weighted Imaging (DWI).
DCE-MRI allows for the evaluation of tumour necrosis and cell membrane permeability. DCE-MRI allows for the assessment of pharmacodynamic endpoints8,9. DWI has been used for the assessment of parameters associated with the rate and distance of water molecule diffusion that may reflect drug access as well as cellular density10.
Multiple pathological studies have shown that lesions assigned to pseudo-progression consist of necrotic tissue and inflammatory cells without evidence of active tumour growth or progressive disease11. Pseudo-progression has been associated with significantly better overall prognosis which led to the hypothesis that it can be considered as a marker of better prognosis and therapeutic response11. Therefore, it is vital to understand as early as possible if pseudo-progression or true progression is present.
Precision Clinical Trial: the Future of Drug Development
Imaging biomarkers derived from advanced MRI and calculated using image postprocessing algorithms offer a unique and powerful insight into the tumour microenvironment in vivo. Carefully designed they can act as surrogate endpoints in clinical trials showing treatment related changes in greater detail earlier than conventional diameter-based approaches. These imaging biomarkers can aid in patient stratification, early scientific efficacy evidence, early go/no-go decisions, adaptive trial design, efficient drug development financing as well as meeting inflection points early. When used adequately in a multi-center clinical trial setting together with centralised reading imaging biomarker reflect scientifically therapy related changes and reduce human error.
References
[1] Miller AB, Hoogstraten B, Staquet M, Winkler A. Reporting results of cancer treatment. Cancer 1981;47:207–14.
[2] Therasse P, Arbuck SG, Eisenhauer EA, et al. New guidelines to evaluate the response to treatment in solid tumors (RECIST Guidelines). J Natl Cancer Inst 2000;92:205–16.
[3] Chiou VL, Burotto M. Pseudoprogression and Immune-Related Response in Solid Tumors. J Clin Oncol. 2015 Nov 1; 33(31): 3541–3543.
[4] Eisenhauer EA, Therasse P, Bogaerts J, Schwartz LH, Sargent D, Ford R, Dancey J, Arbuck S, Gwyther S, Mooney M, Rubinstein L, Shankar L, Dodd L, Kaplan R, Lacombe D, Verweij J. New response evaluation criteria in solid tumours: revised RECIST guideline (version 1.1). Eur J Cancer. 2009 Jan;45(2):228-47.
[5] Hodi FS, Ribas A, Daud A, Hamid O, Robert C, Kefford R, Hwu W, Gangadhar TC, Joshua AM, Hersey P, Weber J, Joseph RW, Zarour H, Dronca R, Gammage L, Hille D, Xue D, Kang P, Chun P, Ebbinghaus SW, Perrone A, Wolchok JD. Patterns of response in patients with advanced melanoma treated with Pembrolizumab (MK-3475) and evaluation of immune-related response criteria (irRC). J Immunother Cancer. 2014; 2(Suppl 3): P103.
[6] Nishino M et al. Developing a common language for tumor response to immunotherapy: immune-related response criteria using unidimensional measurements. Clin Cancer Res. 2013 Jul 15;19(14):3936-43
[7] Seymour L, Bogaerts J, Perrone A, Ford R, Schwartz LH, Mandrekar S, Lin NU, Litière S, Dancey J, Chen A, Hodi FS, Therasse P, Hoekstra OS, Shankar LK, Wolchok JD, Ballinger M, Caramella C, de Vries EG; RECIST working group. iRECIST: guidelines for response criteria for use in trials testing immunotherapeutics. Lancet Oncol. 2017 Mar;18(3):e143-e152.
[8] Workman P, Aboagye E, Chung YL, Griffiths JR, Hart R, Leach MO, Maxwell RJ, McSheehy PM, Price PM, Zweit J; Cancer Research UK Pharmacodynamic/Pharmacokinetic Technologies Advisory Committee. Minimally invasive pharmacokinetic and pharmacodynamic technologies in hypothesis-testing clinical trials of innovative therapies. J Natl Cancer Inst. 2006 May 3;98(9):580-98.
[9] Reynolds HM, Parameswaran B, Roettger D, Finnegan M, Jackson P, Kron T, Siva S; Tumour response assessment on multi-parametric MRI after stereotactic ablative body radiotherapy for primary kidney cancer, ICCR, 2016
[10] Juergens R, Zukotynski K, Singnurkar K, Snider D, Valliant JF, Gulenchyn KY, Imaging Biomarkers in Immunotherapy, Biomark Cancer. 2016; 8(Suppl 2): 1–13.
[11] Nasseri M, Gahramanov S, Netto JP, Fu R, Muldoon LL, Varallyay C, Hamilton BE, Neuwelt EA. Evaluation of pseudoprogression in patients with glioblastoma multiforme using dynamic magnetic resonance imaging with ferumoxytol calls RANO criteria into question. Neuro Oncol. 2014 Aug;16(8):1146-54.
Developing new assets and want to learn more about the use of advanced imaging in your trial?
Contact us directly: info@localhost

Authors
Diana Roettger, PhD, Head of Scientific and Medical Affairs, IAG
Biography: Dr. Diana Roettger, is passionate about the use of medical imaging and computer-based image analysis in strategic drug development. She has 10+ years of experience in medical imaging, algorithm design and computer science. She holds a PhD in computational neuroimaging and has hands-on work experience with world-leading clinical institutions. At IAG Diana directs the development and execution of imaging as a measure of efficacy, eligibility and safety in drug development.
Olga Kubassova, PhD, CEO and Founder of IAG
Biography: Dr. Olga Kubassova is a CEO of IAG, mathematician by background with many years of effective asset positioning and implementing strategies for clinical development, growth, partnering and exit. Her expertise is in identifying and integrating novel approaches to support fast decision making on the efficacy of new therapeutic assets. She broadly leverages her background in computer science, mathematics and imaging to support companies, developing novel technology platforms and decision- making systems. Olga is a renowned healthcare innovator, author of over 40 papers, book chapters and books, speaker and biotech investor, with passion for improving people’s health.